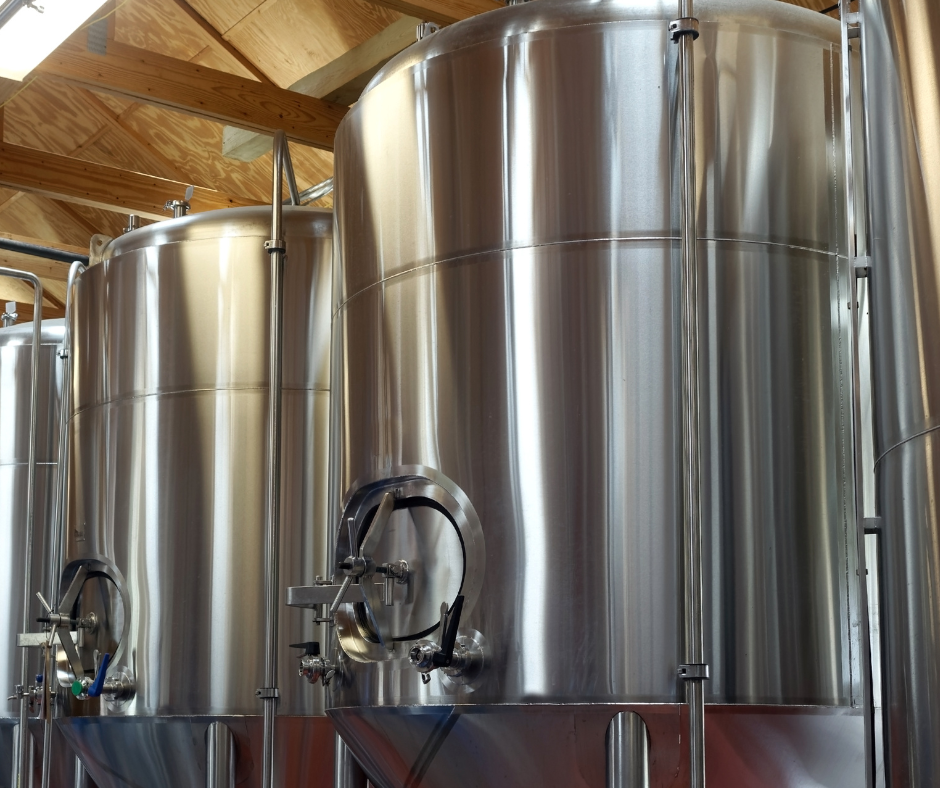
30 Jul How feasible is it to produce large amounts of protein via fermentation?
This Thought for Food summarises the findings of a recently published SNi paper and a new online tool for anyone to explore the implications of large-scale fermentation-produced protein.
An increasing global population raises the challenge of how we adequately feed people in the future without exceeding the capacity of the planet to support food production and all other forms of human activity. SNi research has shown what is needed in terms of nutrition to support populations of the future, but there remains debate on how this food should be produced.
Food production at the global scale has harmful environmental impacts, which has led to important discussion on more environmentally sustainable ways of producing what we need. One technology that has received a lot of attention is the production of food proteins or lipids by “precision fermentation”.
A previous Thought for Food outlined what precision fermentation or fermentation-produced protein is, and the various estimates of its environmental impacts. In short, this technology involves modifying microbes (usually yeasts) so that they produce a desired protein (such as whey protein), and then growing these microbes in large steel vessels and harvesting the protein they produce.
This is a relatively new approach to food production, with only a few commercial companies operating globally, and limited public data about its performance. However, a recent publication in the International Journal of Life Cycle Assessment outlined the process, the important variables in its performance, and its environmental impacts under various assumptions about location and the materials used to feed the microbes.
The SNi team have extended this approach and made the modelling interactive, in a new tool now available on the SNi website. The tool lets users enter a total global production of a target protein, select feedstock, energy generation profile, and adjust fermentation parameters. It then returns the implications of this scale of protein production via fermentation for land and crop use, greenhouse gas emissions, electricity consumption, input costs, and global nutrient supply.
Let’s take an example to see what the model can tell us.
Let’s assume we want to produce an additional 10% of current global protein production per year via fermentation – an additional 18 million tonnes. We’ll set beta-lactoglobulin (a whey protein commonly used as a food ingredient) as the target protein, using sucrose from sugar cane to feed the microbes, with a US energy grid generation profile.
The model calculates how many million tonnes of sucrose would be needed, and subsequently how much sugar cane is needed to produce the sucrose. This gets translated into cropland area required using the DELTA Model® cropland approach: around 11.7 million hectares, 44% of current sugar cane land.
In terms of greenhouse gas emissions, the model calculates a footprint of just under 9 tonnes of CO2-equivalents per tonne of protein produced, around 158 million tonnes of CO2 total. The largest contributors to this are sucrose production and electricity use during the fermentation process, for actions like stirring and pumping.
The electricity required by the fermentation and post-processing is around 150 TWh per year. To make this more tangible, the model tells us this is similar to the total annual electricity production of Ukraine in 2020 – around 1% of total global generation.
The cost per year for the inputs into the process (sugar cane, ammonia, electricity, gas, and water) come in at NZ$102 billion (US$63 billion), which translates to around NZ$5700 per tonne.
From a nutrition perspective, the model reports the 10% increase in protein (as defined by the user) comes with a slightly larger percentage increase in supply of the amino acid lysine, because beta-lactoglobulin is a good source of lysine, and a very minor change in global food energy supply. The published paper also looked at the other macro- and micronutrients, finding negligible changes – this is because sugar cane is not a source of many nutrients, so there would not be much loss of nutrition if this were redirected, and because the protein produced is just that, with no nutrients beyond the amino acid content.
The tool provides a quick and intuitive method to understand the implications of scaled protein production by fermentation. Changing the target protein, the energy generation profile, or the heat source can show you what impact this has on costs and footprints. A knowledgeable user can also edit parameters for the fermenter performance, such as the required purity of the final product, and the mass of microbial cells grown by the end of a fermentation cycle, which all have impacts on the overall results. For those not au fait with these technical details, the default settings represent those in the published literature.
The SNi team built this tool to provide context for the discussion around where novel foods like fermentation-produced protein might fit in a sustainable food system of the future. A simplified version of this tool will soon be included in the DELTA Model®, so that users can add fermentation as a source of protein in complex, global food system scenarios. The ultimate intention is to provide robust, peer-reviewed tools and data to underpin food system decision making.