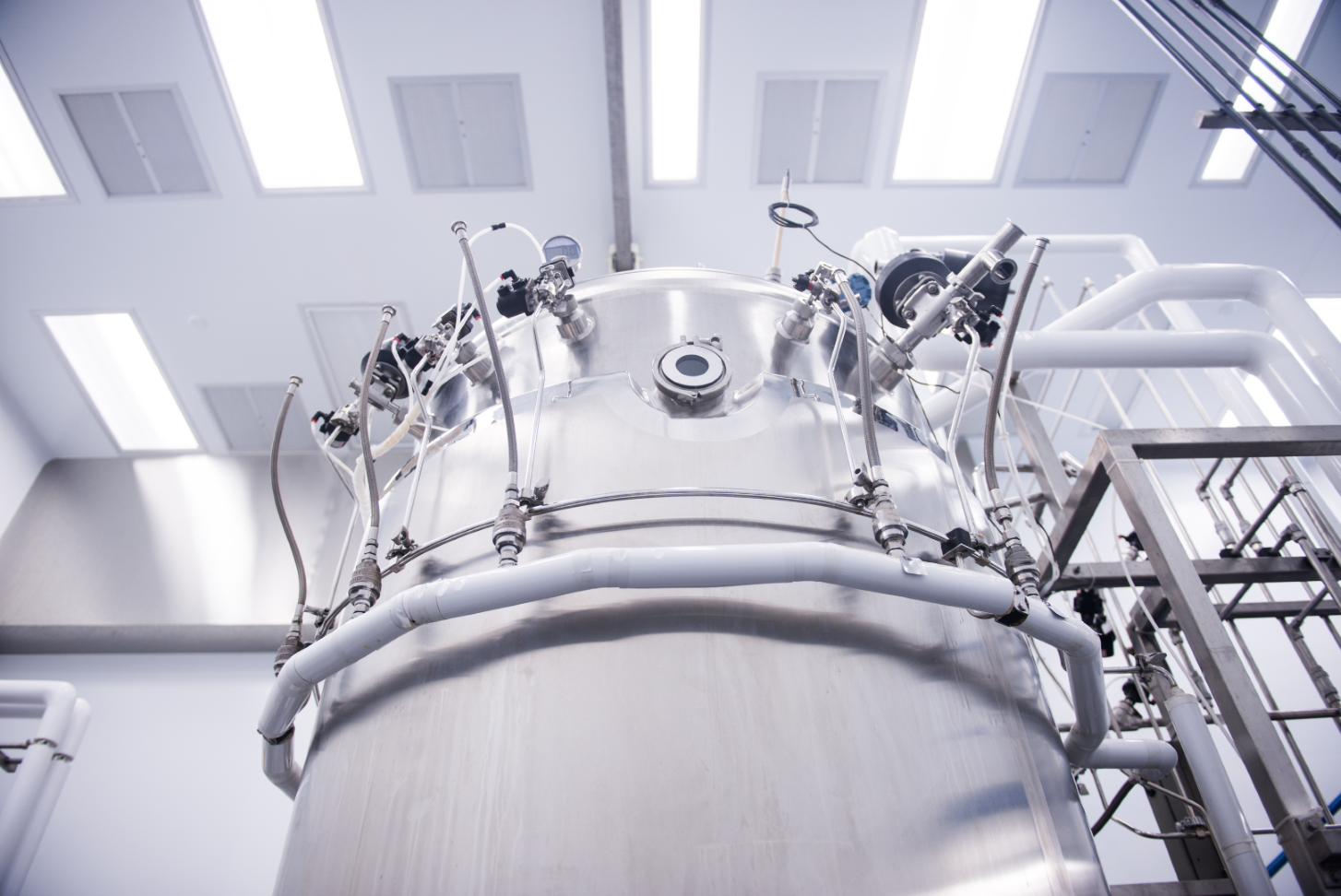
06 Apr Do the environmental impacts of fermentation-produced protein outweigh those of conventional protein sources?
This Thought for Food analyses current knowledge on the environmental performance of fermentation-produced protein using Life Cycle Assessment and compares these impacts with that of traditional animal protein.
Novel technologies in food production are attracting considerable attention and investment. These technologies may be parts of the solution to the pressing issues of ensuring sustainable and healthy food sources for the expanding global population.
Here, we focus on precision fermentation in protein production. Precision fermentation is an approach using microorganisms as “factories” to convert simple sugars into desired food ingredients, such as proteins or lipids, through fermentation within large industrial bioreactors. Its outputs are often sold as individual food ingredients to other businesses to be incorporated into food products for consumers. Fermentation-produced proteins (FPP) are proteins created by applying the precision fermentation technique.
What are the environmental impacts of FPP?
Several previous Thought for Food articles have discussed Life Cycle Assessment (LCA) to help us understand the environmental impacts of food. However, there are few LCA publications or data for FPP. In fact, only two peer-reviewed scientific articles on the topic exist, with a few other commercial reports or student theses available online. This data is essential to support any environmental claims made about the product. So, what can we learn from the limited data that currently exists?
Global warming potential
Greenhouse gas emissions were the most commonly assessed environmental indicator. Most sources claimed that FPP could potentially reduce greenhouse gas emissions compared to conventional animal production. A study by Järviö et al. in 2021 reported that fungal-derived ovalbumin protein production produced 7.5-17 kg CO2 equivalent/kg of product, compared to 26-32 kg CO2 equivalent/kg of product for chicken egg-derived ovalbumin powder. Perfect Day, a start-up company producing protein using precision fermentation, claimed that their whey protein had a 91.2-96.6% reduced carbon footprint compared to the total protein in dairy milk.
However, the work of Behm et al. in 2022 found that the carbon footprint of protein produced via precision fermentation ranged from 5.5 to 17.6 tonnes of CO2 equivalent/tonne of protein, similar to that of extracted traditional milk protein production, which measured 10 tonnes of CO2 equivalent/tonne of protein. The authors stated that sugar production and source of electricity (whether from renewable or non-renewable sources) will strongly influence the overall carbon footprint of precision fermentation. Hence, if sustainable carbon sources, renewable energy, and novel technology were applied, protein production via fermentation could have a lower carbon footprint than traditional animal protein production.
Land use
Cropland need depends on which crop is used to feed the fermentation, and likewise animal land use depends on the animal and the production system (e.g. intensive indoor farming or extensive grazing). FPP also needs land for the production facilities, in addition to cropland. The study of Järviö et al. found that fungal ovalbumin production required less land use than chicken egg white protein production: 4.1-4.5 m2/kg of protein, compared to 36-45 m2/kg of protein for chicken egg-derived ovalbumin. A master’s thesis from Helsinki University in 2023 also stated that the land use required for fermentation-produced protein was less than that for milk. It claimed 4.9 m2 was needed to produce 1 kg of FPP, compared to 273 m2/kg of protein from bovine milk. The land use for protein production via fermentation could be further minimised by using feedstocks such as agricultural byproducts or waste.
Water consumption
Water consumption is similarly challenging to quantify due to the variation depending on crop feedstock and the location of production. Water scarcity footprint is important here, and depends on the availability of water in the location from which it is drawn: high water use is far more of a problem in arid regions compared to temperate regions.
Two contrasting views exist from the papers reviewed about the water use associated with FPP and traditional protein production. Perfect Day whey protein was claimed to consume 73.9 L water/kg of protein, up to 99% less blue water than bovine milk. The thesis study in 2023 found that the water consumption for FPP of 11.5 m3/kg of protein was lower than that for milk of 303 m3/kg of protein. In contrast, Behm et al. reported that the water footprint of FPP ranged from 88-5030 m3 world eq./tonne protein, similar to that of extracted milk protein production at 290–11,300 m3 world eq./tonne protein. If sugar crops used for precision fermentation are cultivated in places with readily available water and energy used in precision fermentation is not derived from water-intensive sources, FPP can have a lower water footprint when compared to dairy milk protein.
Energy demand
It is difficult to come up with a clear comparison between FPP energy use and that of traditional animal agriculture, because so much variation and uncertainty exist. Perfect Day claimed that the energy consumption of FPP was less than that of dairy milk. The company reported requiring 56.3 MJ/kg of protein for Perfect Day whey protein, which was 28.9-59.7% lower than that for total protein in milk. The results of Järviö et al. found that renewable energy demand for fungal protein production of 25 MJ/kg of protein was significantly less than that of chicken egg protein, at 230-250 MJ/kg of protein. wever, the master’s thesis on the topic reported that the total energy demand of FPP and milk were 299 MJ/kg of protein and 185 MJ/kg of protein respectively.
The energy demand depends on sugar production and the details of the fermentation process. Hence, if renewable electricity and sustainable sources of sugar were used, there could be a potential reduction in energy requirements of FPP compared to traditional animal protein production.
Putting together diverse conclusions
To sum up, by switching to sustainable sugar production, renewable electricity and innovative production systems, protein production via precision fermentation could have lower environmental impacts than animal-derived proteins.
However, FPP provides a single nutrient, namely protein, while the animal-sourced foods used as a comparison offer a diverse array of essential nutrients crucial for human nutrition, in addition to protein. These include carbohydrates for energy, fats essential for bodily functions, vitamins necessary for metabolic processes, and minerals vital for overall health. The global population requires complete, balanced nutrition, therefore the formats and combinations in which FPP is ultimately consumed are of critical importance.
Furthermore, FPP in food production is an emerging concept with limited available data. To reiterate, there have been only two peer-reviewed articles on its environmental impact. Most cellular agriculture research is based on findings that are not peer-reviewed and mainly from vested interests. Thus, additional peer-reviewed research is necessary to fully understand the impact of precision fermentation on the environment. Some of the questions for future research could be:
- Could recycled waste products from fermentation serve as a sustainable feedstock to mitigate the environmental footprint?
- How can energy-efficient fermentation technologies, renewable energy sources, and sustainable carbon sources serve as integrated methods to reduce the environmental footprint of protein fermentation?
- Would FPP production come out on top when conducted on a large commercial scale?
To help in understanding these questions, the Sustainable Nutrition Initiative® team have developed an interactive model to understand the inputs and outputs of FPP when scaled to be a significant proportion of global protein supply. Watch this space for an upcoming standalone FPP model and integration of this work into the DELTA Model®.
This Thought for Food was written by Quinn Vy, a summer intern, with the support of the SNi team.